The Intricate Dance of Nuclear Fission: A Closer Look at Atom Splitting
The term “atom” traces its roots back to Latin, meaning indivisible. However, this seemingly solid concept is shattered when we delve into the realm of nuclear fission.
Recent simulations conducted by US theoretical physicists have unveiled the microscopic intricacies of the moment an atom undergoes division, shedding light on a phenomenon that has revolutionized science and technology.
In the year 1938, physicists Otto Hahn, Lise Meitner, and Fritz Strassmann shattered the notion of atom indivisibility by demonstrating how uranium nuclei split when bombarded with neutrons.
Despite its widespread applications in warfare, energy production, medicine, and scientific research, nuclear fission remains a complex and enigmatic process.
Going beyond the simplistic image of protons and neutrons clustered together like gumballs in a dispenser, the nucleus of a large atom is a chaotic whirlpool of quantum activity.
Understanding the behavior and interactions of individual nucleons within an atom is a formidable task, especially when dealing with atoms undergoing significant transformations.
To simplify the process, theoretical physicists from Los Alamos National Laboratory and the University of Washington have broken down the fission process into four distinct steps.
Initially, within the first 10^-14 seconds, a slow-moving neutron causes the nucleus to bulge and reconfigure itself into a saddle point, resembling a tiny peanut shell.
This is swiftly followed by the saddle-to-scission phase, lasting around 5×10^-21 seconds, where the fragments of fission are established.
The third step, occurring in a mere 10^-22 seconds, involves the scission or neck rupture, marking the official separation of the nucleus.
Finally, in the last step taking approximately 10^-18 seconds, the fission fragments realign themselves and accelerate away, releasing neutrons, gamma rays, and triggering other decay processes.
Various theories attempt to elucidate the migration of subatomic particles during the fission process, yet experimental results often defy basic assumptions or clash with microscopic models of proton-neutron interactions.
Utilizing a framework developed by lead author Aurel Bulgac from the University of Washington, a quantum many-body simulation has provided the most precise depiction of the scission moment, where the bridge connecting the two halves of a large atomic nucleus snaps apart.
This simulation, conducted on uranium-238, plutonium-240, and californium-252 under different conditions, required extensive computational resources from the US Department of Energy’s Oak Ridge National Laboratory supercomputer.
Bulgac emphasizes the accuracy of their theoretical description of neck rupture, devoid of assumptions or simplifications, offering a specific prediction based on established equations of motion and quantum mechanics.
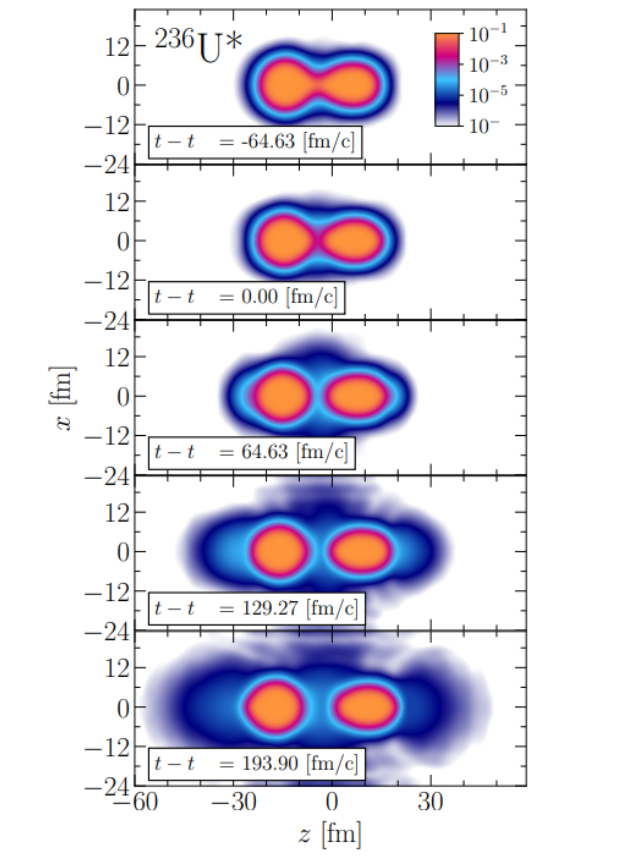
The simulation uncovered surprising details in the fission process, debunking previous models that predicted quantum randomness in neck rupture. Instead, a distinct density “wrinkle” of subatomic particles preceded the scission point.
Furthermore, the simulation highlighted a discrepancy in the timing of division between proton and neutron necks, with the proton neck breaking first before the neutron neck.
Significantly, the model confirmed the emission of highly energetic neutrons during the scission stage, predicting their energies, angular distribution, and escape directions.
Bulgac notes that experimental validation is crucial to verify these novel insights into the atom’s splitting process.
This groundbreaking research was published in Physical Review Letters.